METHODOLOGY
FT-IR spectroscopy can be used for
combustion monitoring in several ways. A sample can be extracted from any point in the
furnace or stack using a probe. This sample can then be preconditioned (removal of water
or particles, dilution with air) and analyzed in a gas cell at a user specified path
length using absorption spectroscopy. Alternatively, in-situ measurements can be made
along a line-of-sight through the furnace or stack using either absorption, emission or
combined absorption/emission spectroscopy.
Measurements of gas concentration are
based on the absorption of radiation from an infrared source. Temperature can be obtained
from gas band shapes (which reflect the population of vibrational and rotational energy
levels) in absorption or in emission. Temperatures can also be obtained from a combination
of emission and absorption. Particle size is obtained from radiation scattering by
diffraction. Tomographic reconstruction of spectra for multiple lines-of-sight can be used
to obtain spatially resolved spectra, from which analysis of temperature and concentration
may be performed. Several of these measurement methods have been successfully employed in
commercial industrial plants under harsh conditions.
RESULTS
FT-IR Line-of-Sight Transmission Analysis in a Smoke Stack of a
Pulp and Paper Mill Recovery Boiler
Transmission measurements were made
across a 5 meter diameter smoke stack of an operating recovery boiler. Results for the
quantitative analysis are presented in Table 1. Most species can be observed at the ppm
level, even with the high concentrations of water. The uncertainties for these
measurements are mainly due to the high water concentrations. Similar quantitation
performed on spectra with no water have extremely low uncertainties for the gases in the
table (< 0.05 ppm). The concentration of NO and NO2 were in good agreement
with the total NOx concentration, determined by an extractive measurement. The negative
value for CH4 indicates that there was more CH4 in the air outside
the stack (where the reference was taken) than in the stack itself.
Spectrum
Quantitative Technique |
Concentration
(PPM) |
CO |
15.7 +\-0.1 |
NO |
54.8 +\-4.5 |
NO2 |
6.0 +\-0.09 |
NH3 |
-1.1 +\-0.4 |
HCl |
1.7 +\-0.3 |
CH4 |
-5.8 +\-0.5 |
SO2 |
9.8 +\-8.0 |
Table 1:. Gas concentrations
from a Black Liquor Recovery Boiler.
To improve quantitation in the presence
of interfering species, AFR has developed a method which employs a library spectrum
and a library perturbation spectrum which includes features which vary with pressure,
concentration or temperature. Figure 1 shows time lines for the quantitation of CH4, CO2,
CO, and Water.
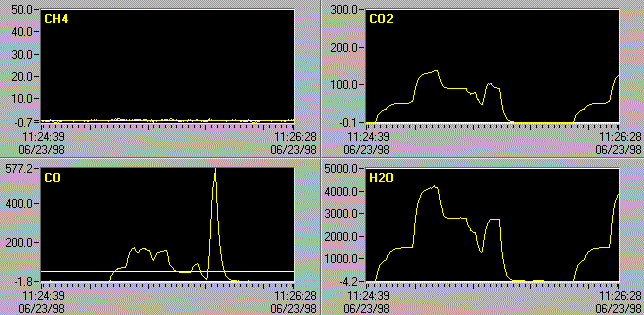
Figure 1. Computer screen
showing effect of quantitative algorithm applied to 10 ppm of NO in the presence of 1000 -
10000 ppm H2O in 1 atmosphere of air at 30°C. a) Single H2O
reference, b) H2O reference and perturbation reference.
FT-IR Emission/Transmission
Measurements in a Pulp and Paper Mill Recovery Boiler
Absorption and emission measurements
were made directly across a black liquor recovery boiler itself. Typical results are
presented in Fig. 2. Although the eye cannot see through the fume because of scattering in
the visible region of the spectrum, Fig. 2a shows that the stream is not opaque to the IR
beam. The spectrum contains opaque regions because of the absorptions by CO2
(2350 cm-1), H2O (3500 cm-1 and 1600 cm-1),
and the fume (1200 cm-1). The absence of a strong absorption near 2143 cm-1
(the CO region) indicates that the boiler is operating fairly efficiently.
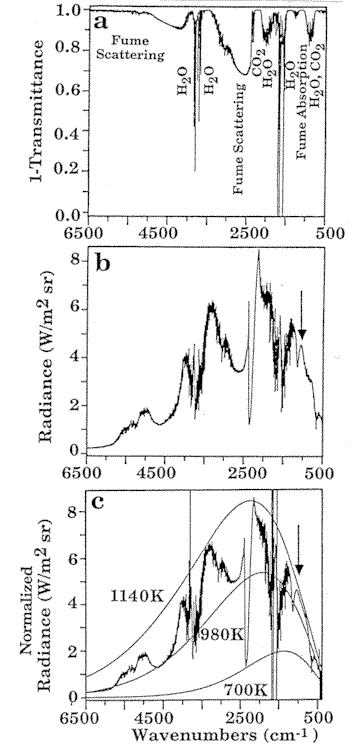
Figure 2. Tests of an
FT-IR
monitor on the recovery boiler of a paper mill revealed that for sub micron fume
particles, scattering decreased rapidly with decreasing wavenumber (a), creating partially
transparent windows in the spectrum. The experiment also demonstrated that a set of
temperature measurements could be derived from the radiance spectrum (b) and from the
normalized radiance (c) with a gas temperature of 1140 K, wall temperature of 980 K, and
cold gas temperature of 700 K.
A set of temperature measurements can
be derived from the radiance spectrum (Fig. 2b) and the normalized radiance (Fig. 2c). The
sharp notch in the radiance near 2400 cm-1 is due to cold CO2
outside the boiler absorbing the hot CO2 radiation. However, at both edges of
the band, the radiation from hot CO2 is not absorbed by the cold CO2,
and these regions can be used to determine the hot CO2 temperature. Figure 2c shows that
several temperatures are present. The 1140 K black body curve fits the gas absorptions for
CO2 and H2O, and the fume absorption. This is the gas temperature
inside the furnace. The 700 K curve fits the cold CO2 band and identifies the
temperature near the boiler optical access port. The radiance at high wavenumbers is due
to fume scattering of the wall radiation. We find that a temperature of 980 K (Fig. 2c) is
in agreement with the estimated wall temperature.
The fume particle size can be derived
from the absorption spectra. By fitting the fume absorption and scattering derived using
Mie theory, one finds that the 0.2 µm particle size is incorrect because the fume
absorption features are too large to be consistent with the observed scattering spectrum.
Similarly, a 0.6 µm particle size is also incorrect since it predicts absorption peaks
that are too small to fit the measured scattering spectrum. The best fit is clearly a 0.4
µm particle size in agreement with measurements on captured fume particles.
FT-IR Measurements in Power Plants
Both in-situ and extractive FT-IR
measurements were performed during field tests at a Cogentrix coal-fired cogeneration
plant (Richmond, VA). Urea injection is used at two locations in the furnace to control
NOx formation.
For extractive FT-IR measurements, the
flue gas was sampled prior to the lime spray dryer, using an STI Dilution Probe
(Graseby/STI, Waldron, AR). In general, the FT-IR measurements were in reasonable
agreement with those of the resident CEM systems.
In-situ FT-IR spectra were collected
across a 2.34-meter duct directly after the economizer section. The operating conditions
at this site were very harsh; the ambient temperature was in excess of 73°C (110°F) and
vibrations were significant. In spite of this, the system performed well for three full
days of testing. Both NH3 and HCl were measured, and were consistent with
changes in the boiler operating conditions. Clearly, the ability to determine NH3
concentrations on a short time scale (< 30 seconds) will be very useful in the control
of urea injection used for NOx reduction.
Tomography
For non-homogeneous samples,
measurements of emission and transmission will yield an average across the line-of-sight.
Alternatively, it is possible to employ tomography to obtain spatially resolved data.
Tomography refers to the construction of a three dimensional image by stacking up
two-dimensional slices of the image. The two-dimensional images can be mathematically
"reconstructed" from line-of-sight measurements across the object. We have
employed tomography for both transmission and emission spectra for an ethylene diffusion
flame.
For the absorbance spectra, a Fourier
reconstruction of the two-dimensional images from the projections has been performed. For
radiance, the spectra were corrected for self-absorption. The Fourier reconstruction
technique was then applied to these corrected radiation spectra to give local radiance.
The analysis of these local spectra has
been performed in the same manner as for thin homogeneous samples as described above.
Plots showing the concentrations and temperatures in the center plane of the flame are
presented in Figs. 3 and 4. A photograph of the flame is shown alongside reconstructed
images (color scale) of organics, soot, CO2, and H2O. FT-IR
tomography has thus demonstrated the unique capability of measuring local species
concentrations and temperatures in mixed phase systems.
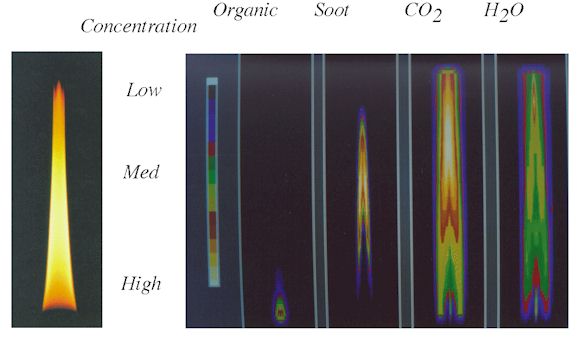
Figure 3. The
Ethylene Diffusion Flame with Central Plane Images of Relative Concentration Reconstructed
from FT-IR Transmission.
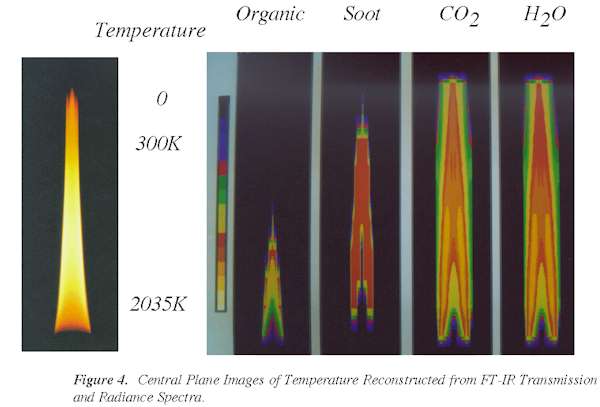
Figure 4. Central
Plane Images of Temperature Reconstructed from FT-IR Transmission and Radiance Spectra.
