The system can also provide measurements of radiance as well as
directional-hemispherical reflection and transmission over a wide spectral range
from 12,500 to 500 cm-1 (0.8 to 20 microns) for the Model 205 WB, and
from 6,000 to 500 cm-1 (1.7 to 20 microns) for the Model 205 NB.
The Model 205 WB Emissometer has additional
extended capabilities to operate as a stand-alone FT-IR spectrometer covering
the Near and Mid-IR spectrum from 12,500 cm to 500 cm-1 with up to 1
cm-1 resolution. The Model 205 NB covers the Mid-IR spectrum from
6,000 to 500 cm-1 .
The Series 205 Emissometers significantly advance
the state-of-the-art in emissivity measurements. Previous methods and
instruments for measuring spectral emittance at elevated temperature required
the precise knowledge of the sample temperature. The Model 205 overcomes these
difficulties and provides all information necessary to simultaneously
determine the precise temperature and emissivity for the same target spot on the
sample.
Optics
The Emissometer is shown schematically in Fig. 2. All optical components,
including the FT-IR spectrometer, are mounted on a 3 foot x 4 foot optical
bench. The hemi-ellipsoidal mirror enables the measurement of radiation in a
hemispherical-directional mode. The sample can be heated with an oxy/acetylene
torch, CO2 laser, or other means. The FT-IR spectrometer is utilized
in the emission mode and can accept radiation from either side of the sample by
positioning the selector mirror. The design of the spectrometer's interferometer
allows for the incoming beam to be modulated and split into two outgoing beams.
In the Model 205 WB Emissometer, two separate detectors are utilized to measure
near and mid-IR energy in these two beams simultaneously. A room temperature
indium-gallium-arsenide detector is used for the Near-IR (12,500 to 6,000 cm-1),
and a liquid nitrogen cooled mercury-cadmium-telluride detector is used for Mid-IR
(6,000 to 500 cm-1). For the Model 205 NB Emissometer, only the MCT detector is
required.
The hemi-ellipsoidal mirror has both
foci inside the mirror. A near-blackbody source is located at one of the foci
and the sample is located at the other focus. This mirror geometry, combined
with the radiating characteristics of the near-blackbody source, provides a
means of measuring the hemispherical- directional reflectance of the front
surface of the samples. Likewise, for transmissive samples, the hemispherical
-directional transmittance can be measured from the back side.
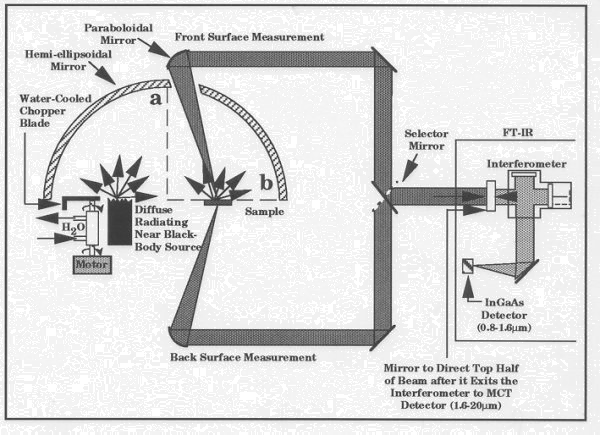
Figure 2. Schematic of Benchtop Emissometer.
Operation
An integral part of the optical system is the rotating chopper system which
moves either an aperture or a cold near-blackbody element in front of the
source. The FT-IR data collection system is synchronized with these two states,
and allows for the distinction of sample radiation from reflected/transmitted
radiation as follows. For the reflectance measurement, the IR beam originates at
the near-blackbody
source at one focus of the hemi-ellipsoidal mirror. The radiation reflects from
the hemi-ellipsoidal mirror and is focused onto the sample at the other focus
where it is reflected (scattered) by the sample into the interferometer. The
reflectance and the sample radiance are measured together when the aperture on
the chopper rotor is in place over the source (chopper open condition). This is
shown by the top curve in Figure 3. When a cold near-blackbody is substituted
for the aperture over the source (chopper closed condition), it is the sample
radiance alone which is measured (bottom curve in Fig. 3). Both the radiance (r)
and directional- hemispherical reflectance (R) can be obtained from these two
spectra. Transmission measurements (T) are similarly obtained by repositioning
the selector mirror.
The bidirectional scanning ability of the
spectrometer's interferometer allows collection of sample radiance (chopper
closed) in the "forward" scan, and sample radiance plus reflectance
(chopper open) in the "reverse scan". At 32 cm-1 spectral
resolution, a complete forward and reverse motion of the interferometer is
accomplished in ~0.5 seconds, corresponding to a chopper rate of 2 Hz. Signal
processing automatically separates forward motion scans from reverse motion
scans and allows for signal averaging from sequential collection of data for
each motion. Typically, a data set consists of 16 co-added scans of each
component of the front surface measurement. For non-opaque samples, the selector
mirror is flipped and 16 co-added scans of each component of the back surface
are collected.
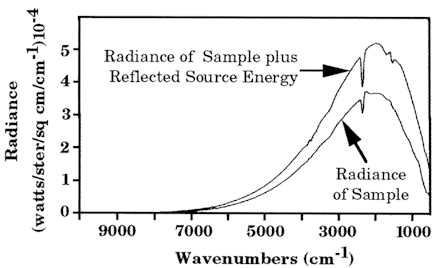
Figure 3. Spectral Measurements Performed with the Emissometer.
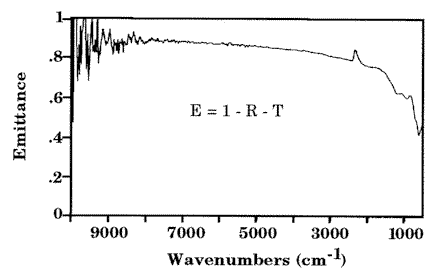
Figure 4. Determination of Spectral Emittance from the Measured Hemispherical
Reflectance (R) and Hemispherical Transmittance (T).
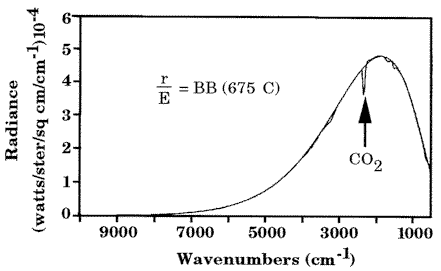
Figure 5. Determination of Temperature by Matching (r/E) with a Blackbody Curve.
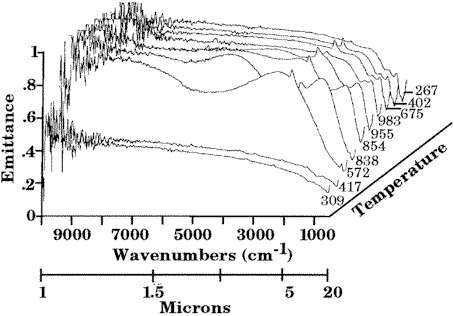
Figure 6. The Radiative Property Change of a Metal Alloy as it is Heated in Air.
Once the spectra are acquired, Spectral emittance (E) of the hot sample is
determined by conservation of energy: E = 1 - R - T (Fig. 3). The precise sample
temperature is simultaneously determined by the Planck "Blackbody"
(BB) relationship: r/E = BB. Hundreds of spectral data points or
"colors" are used tomatch the shape and amplitude of the Planck temperature curve as shown in Figure
4. As shown in Figure 5, the emissometer can rapidly monitor spectral emittance
as a function of temperature and time.
Samples
Samples are mounted into position with a clamping devices which is arranged so
as not to contribute extraneous radiation into the measurement. Three
intersecting visible lasers beams are utilized to ensure correct positioning of
the sample. Sample sizes on the order of 1 to 1-1/2 inches square or diameter
are convenient, although samples as small as 1/4" square have been
measured. The
measurement spot on the sample is variable from 1 millimeter diameter to 3
millimeters in diameter. Samples have been heated by a variety of methods. These
include: i) torch flames (propane, hydrogen, oxy/acetylene), ii) focussed
infrared radiation from high intensity lamps, and iii) infrared radiation from
a CO2 laser (25 W, continuous wave). Sample thickness of less than
1/4" is con-venient for sample heating in order to achieve uniform sample
surface temperatures when heating on the back surface. Torch heating allows the
sample to be heated from the back, the front, or both surfaces. The influence on
the measurements due to the radiation contributions from the combustion products
of a torch flame is not a problem as it is limited to narrow spectral regions.
Specifications